Module 5 GPS and Wayfinding
5.1 Preliminaries
5.1.1 Readings
Readings should be done when referenced during this week’s lesson. Do not read before starting the lesson.
5.1.2 Learning Objectives
By the end of this lesson, students will be able to:
- Explain how GPS works
- Give three examples of how GPS is used today
- Define three types of wayfinding tasks
- Describe how GPS has changed our wayfinding abilities and behaviours
Activities for Module 5
- Readings
- Assignment A-M6
- Quiz Q-M6
5.2 What is GPS?
You have no doubt heard the three letters GPS before, and perhaps even know what it stands for - global positioning system. For a technology that is largey associated with space: locating positions on the earth’s surface, navigation, tracking, etc. it is a little ironic that GPS is actually all about time. One of the early inventors of GPS was Roger Easton who worked for the US Naval Research Laboratory. In early 1960s Roger was experimenting with long-range bi-static radar systems and needed a way to synchronize the clocks for the two radar stations. Any two clocks will eventually drift out of sync - and resolving the discrepancy between the two clocks is a problem known as clock synchronization.
Roger ended up using a highly accurate clock called an atomic clock (aka cesium beam clock) to synchronize the two clocks by driving the 100 mile distance between the two stations. This was a rather inefficient method of resolving the time synchronization issue for the radar system, and eventually led to the idea that an atomic clock on a satellite could broadcast time corrections to the two radar stations simultaneously, synchronizing the clocks and saving on gas in the process.
Satellite-based clock synchronization was the fundamental idea underlying the development of GPS technology. With such a clock onboard a satellite, the sending and receiving time of the signal could be determined, and given that the signal is travelling at a constant speed (theoretically) - the speed of light, the time could be converted to a distance. Let’s take a closer look at this idea.
5.3 How GPS Works
We start with a ground-based receiver receiving a signal broadcast from a satellite. The satellite is continuously broadcasting its atomic clock time. This time is converted to a distance - given that \(distance = speed \times time\) and we know that light travels at around 300,000 km/s, if a signal sent by the satellite might take 0.07 seconds to arrive at the receiver, we could easily see that \(300,000 km/s \times .07 s = 21,000 km\), which is about where a GPS satellite sits in orbit when directly overhead. Try plugging these values into a calculator and see how they work out. Now if that satellite is further along its orbital path in the horizon, that time value will be larger, and the distance will increase accordingly.
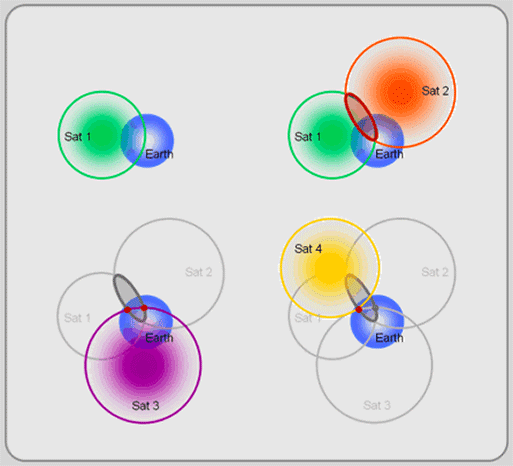 Image taken from GIS Commons. Licensed under Creative Commons (CC SA 3.0).](images/2.141.gif)
Figure 5.1: GPS satellite trilateration. Image taken from GIS Commons. Licensed under Creative Commons (CC SA 3.0).
What we know at that point is that the receiver is 21,000 km from the satellite. We can envision this as being on the surface of a sphere where the satellite is at the centre, and the spherical radius is 21,000 km. Since we could be located anywhere on this hypothetical sphere, it’s not enough to determine our location. Imagine we connect to another satellite, and determine our distance from that satellite is 27,000 km. At this point, we know we are located on the surface of two theoretical spheres, and in fact two such spheres will intersect along a circle, so we have to be located somewhere on the circle where the two spheres intersect.
If we add a third known distance my connecting to yet another satellite, we can now be located at only two possible locations, where all three spheres intersect. If we have an approximate idea of where we are (e.g., if one is on earth and one isn’t), we can likely discard one as incorrect, or if we add more satellites we can then determine our location exactly.
This is how GPS works, via a constellation of satellites broadcasting time information, which is then received by GPS receivers in your phone or on your handheld GPS device to determine your exact location. The GPS system that was launched by the US as the first global navigation satellite system called NAVSTAR GPS was comprised of a global constellation of 24 satellites.
](images/ConstellationGPS.gif)
Figure 5.2: GPS constellation Image source: WikiCommons
In the figure above the blue point represents a GPS receiver. As the earth rotates on its axis and the GPS satellites move along their orbits, the receiver can connect to a different number of sensors. Note as above, we only need 4 satellites to detect our location on earth, but due to a variety of sources of error, we typically want more satellites to determine an accurate location.
](images/image_gallery.jpeg)
Figure 5.3: Artist’s view of the deployed TIMATION-1 satellite (Original image credit: NRL) Image source: https://directory.eoportal.org/
Returning to the early development of the GPS program by the US, Roger Easton’s satellite-based clock synchronization scheme was tested in a program called TIMATION, which launched and operated two small satellites in 1967 and 1969 to demonstrate the concept of using synchronized clocks that provided passive ranging signals. TIMTATION was one of three competing satellite based programs (TIMTATION, 621B, and TRANSIT) which in 1973 were integrated into one program for satellite-based navigation called NAVSTAR GPS.
NAVSTAR GPS launched several satellites into orbit over the next decades while refining the developing the space-borne satellite technology and the control segment responsible for signal processing, sending commands and data to satellites, overall maintenance and monitoring of satellites.
In 1994 the 24th GPS satellite was added to the system providing truly global coverage for navigation and positioning. The development of GPS as a military technology had clear applications in navigation, identifying targets, and many other uses for naval, air force, and army operations. However the civilian applications of GPS which we depend on today were more of an afterthought. Once GPS was up and running as military technology, civilian use was eventually enabled, however the positioning accuracy was intentionally degraded for non-military uses through a process called selective availability. Thankfully, selective availability was disabled by the US Government in 2000, ushering in an era of high-accuracy satellite-based navigation and positioning for a wide array of civilian and commercial uses.5.4 Wayfinding
GPS data makes up a significant component of human-oriented Digital Earth data. Much of the VGI and location-based services discussed last week depend on accurate and ubiquitous positioning information. GPS technology has become so ubiquitous we expect to be able to find our way wherever we are on earth. If you are dropped down into a random location anywhere on earth, you would probably expect to open your phone, see your position, and see some geographical context data that would help you to figure out where you were and where to go. Let’s try it:
Stop and Do - 1
Go to this site that generates random geographic coordinates and click Pick Coordinates. Note; since the earth is 70% covered by water you may have to click a few times to find on a spot on land. On the first land spot you find, zoom in as much as you can to see the area. Expand the view to full screen (upper right corner) so you can get a feel for the area. Then slowly zoom out and make a plan for how you would get home if dropped in that location.
Assuming you had this map and your location pinned on the map, how would you find your way to get home?
Now, zoom in or out until the scale bar at the bottom of the map says 5 km. Then click Pick Coordinates until you find another land spot. Once you do, try to figure out where you are. Look for clues. What information are you looking for to help you understand your geographic context? Landscape type, place names, are there geographic borders or street names? If you can’t see anything, zoom out one level. Once you are fairly certain of where you are, make a note of what the scale bar says (e.g., 5 km, 10 km, 100 km, etc.)
 Image retrieved from Wikimedia Commons. Licensed under CC BY-NC-SA 2.0](images/phone.jpg)
Figure 5.4: Distracted wayfinders in a common urban scene. Image retrieved from Wikimedia Commons. Licensed under CC BY-NC-SA 2.0
5.4.1 Characteristics of Wayfinding
The process of finding your way is part of a field of study known as wayfinding. Wayfinding can be defined as goal-directed movemennt to a distant location that cannot be directly perceived by the traveller. Thus, wayfinding is an extremely common human activity. You may ask why is this a topic worthy of scientific study?
It turns out that there are extensive individual differences in wayfinding abilities. On average, men tend to do better than women, and younger people tend to do better then older people. Disorientation and inability to navigate is also associated with Dementia symptoms and people with Alzheimer’s disease. But these skills are essential to our understanding and experience of the world.
Why then does wayfinding apply to our understandign of the DE?
There are two primary reasons. Firstly, the widespread adoption of GPS and assisted navigation aids have led to declines in traditional wayfinding and spatial cognition skills. Secondly, understanding the nature of human wayfinding in natural environments may shed light on how people navigate in digital environments.
Much research has explored wayfinding in digital worlds (e.g., computer gaming and/or RPG’s), and as we develop new immersive DE applications, wayfinding through digital worlds constructed of geospatial data may soon be a reality.
Wayfinding can be classified into three types of tasks.
- Goal-directed movement to reach a familiar destination
- Exploratory movement with the goal of moving back to a known location
- Goal-directed movement to reach a novel destination
You can think of examples of these that you engage in every day. Commuting to school or work is movement towards somewhere you already know (task 1) is a different wayfinding activity from trying to find your hotel from the airport when you travel to a new city (task 3). Once you get to the hotel and get comfortable, you might head out to explore your surroundings, with the intention of ultimately coming back to your hotel (task 2).
Take 30 minutes and watch this video (28:31) with the author of a recent book investigating wayfinding.
Stop and Do - 2
Describe a time when you arrived in a new location and had to find your way. How did you do it? If you used your phone for navigation, reflect on what you would have done differently in this situation had you not had this device?
5.5 Ethical Issues of GPS Tracking
In a world where so much of our lives are tracked and encoded as digital transactions, increasingly our geographic location is either explicitly or implicily revealed through our use of apps and location-based services.
The notion of geoprivacy has been introduced in the academic literature to encompass the need to preserve peoples right to their location data and information about their movements. As data are filtered and processed through more complex systems of apps, services, and related data processing tools many of which are owned and exist for commercial profit, the control of one’s own location data becomes difficult. GPS tracking data have enormous value to companies to
- reveal human movement patterns to help them target advertising,
- to governments hoping to inform planning and management of public places,
- as well as to law enforcement agencies when seeking to identify the whereabouts of a person at a given time.
The use of GPS tracking data for these purposes is only possible when people either explicitly consent to the use of their location data by third parties, or as is more common, people have no agency to control how their data are used after is collected.
Are you concerned about preserving your geoprivacy when you use location-based apps and services? Why or why not?
5.6 Summary
The evolution of GPS from its early days in the 1970s to the current state of the art has transformed how we create geospatial data - but also how we move within and interact with geographic space. As we have seen, wayfinding and navigation is a critical human skill with links to memory, identity, and ultimately what it means to be human. As more of our navigation and wayfinding tasks are coupled to GPS-enabled devices, there may be important information we are missing about coming to know our surroundings. The links between the technological revolution of GPS and the changes in how we navigate are still being discovered.
5.6.1 Key Terms
- gps
- clock synchronization
- gnss
- selective availability
- wayfinding
- geoprivacy